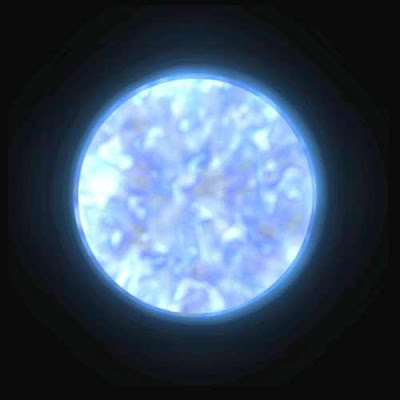
Artist’s impression of LS IV – 14 116. The white clouds are rich in zirconium and lie above the blue surface of the star. Image: Natalie Behara
By Royal Astronomical Society, United Kingdom.
Published: December 8, 2010.
A team of astronomers led by graduate student Naslim and supervisor Simon Jeffery from Armagh Observatory in Northern Ireland has found what at first sight appears to be the most zirconium-rich star ever discovered. Zirconium, the material used by jewelers to make false diamonds, glitters in clouds above the star's surface.
The team made the discovery while looking for chemical clues that explain why a small group of stars reaching the end of their lives, known as helium-rich hot sub-dwarfs, have less hydrogen on their surfaces than other similar stars. Using data obtained with the Anglo-Australian Telescope at the Siding Spring Observatory in New South Wales, the team looked at the evolved star LS IV-14 116, which is 2,000 light-years from the Sun in the direction of the border between the constellations Capricornus and Aquarius.
The scientists used the telescope instruments to disperse the light of the star into a spectrum. Different elements and molecules give rise to characteristic patterns in stellar spectra, allowing Earth-based scientists to determine their composition.
As expected, the spectrum of LS IV-14 116 had the usual lines arising from more common elements, but other strong lines were less easy to identify. A careful study showed four of these lines were due to a form of zirconium that only exists at temperatures above 36,000° Fahrenheit (20,000° Celsius) and that had never previously been found in an astronomical spectrum.
Alan Hibbert, from Queen's University Belfast, computed a model of the zirconium atom to predict the expected line strengths. With this information, the team measured the zirconium abundance in LS IV-14 116 to be 10,000 times as high as in the Sun — meaning that 1 atom in every 200,000 is zirconium rather than 1 in 2 billion. Further work showed the remaining unidentified lines to come from strontium, germanium, and yttrium. These elements are found to be between 1,000 and 10,000 times more abundant than normal.
The Armagh team argued that the unusual abundances in LS IV-14 116 are caused by the formation of cloud layers in the star's atmosphere — the only part of a star that can be seen directly. High concentrations of certain elements, mainly metals heavier than calcium, build up in these clouds, but the same elements are scarce in layers above and below, meaning that their overall abundance is near normal. Natalie Behara, now at the University Libre de Bruxelles, calculated models of the star's atmosphere. This may well have a dramatic appearance, with many thin cloud layers, each due to a different metal.
The team also suggests that the star is shrinking from being a bright cool giant to a faint hot sub-dwarf. As the star shrinks, different elements sink down or float up in the atmosphere to a region where they become highly visible, making the apparent composition sensitive to the star's recent history.
Most stars like the Sun have about 10 zirconium atoms for every million silicon atoms. LS IV-14 116 has 2 million zirconium atoms for every 1 million silicon atoms. It is estimated that the zirconium layer seen in LS IV-14 116 would weigh about 4 billion tons, or 4,000 times the world's annual production of zirconium.
"It was very exciting to discover these completely new chemical signatures in our data,” said Jeffery. “The peculiar abundances measured in this star, and hopefully in others, offer a new tool to explore a stage of stellar evolution that is extremely difficult to observe directly."
"The huge excess of zirconium was a complete surprise,” said Naslim. “We had no reason to think this star was more peculiar than any other faint blue star discovered so far."